Our Research Engineered Nitrogen Fixation: Expression in plant organelles
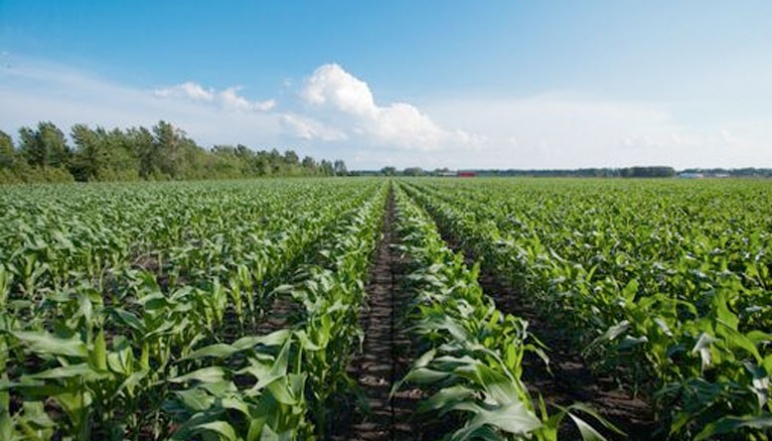
Photo Credit: iStock Images
Principal Investigator
Christopher Voigt
- Daniel I.C. Wang Professor of Advanced Biotechnology
- and department head
- Department of Biological Engineering
Christopher Voigt, PhD is the head of the Department of Biological Engineering at MIT. He is an expert in synthetic biology and his lab has applied genetic engineering to problems in agriculture, health, computation, medicine, chemicals/materials, national security, and infrastructure. He holds the Daniel I.C. Wang Professorship and is Co-Director of the Synthetic Biology Center. He received a BSE in chemical engineering from the University of Michigan (1998) and a PhD in biophysics from Caltech (2002). He is a founder of Pivot Bio (microbial agricultural products), Asimov (human cell synthetic biology), and Fieldstone (national security and biotechnology).
Challenge:
How can we enhance the yield of cereal grains without the use of chemical fertilizer?
Research Strategy
- Engineer a method to biologically transfer the nitrogen-fixing capability of legumes to cereal grains via re-programmed plant cell mitochondria
- Initiate an international collaboration of leading scientists (Spain, Germany, and MIT) to speed discovery and development
Project description
Plants need nitrogen to grow. Globally, the primary food crops are cereals (rice, corn, wheat). They are not able to fix their own nitrogen, but in nature, they obtain microbially derived nitrogen from soil. However, because of the density of modern agriculture, they would quickly leach the nitrogen and yields would collapse. This is avoided by adding chemically derived nitrogen. In contrast, legumes have special associations of nitrogen-fixing microbes in specialized organs, called nodules. As such, they do not need added nitrogen. The dream of biotechnology has been to move this capability into cereals so that they do not require added nitrogen. The challenge has been that nitrogen fixation requires the simultaneous transfer of many genes that must be carefully balanced in order to obtain activity. There are also biochemical constraints, including oxygen inactivation, complex metal cofactors, and high energy and redox potential requirements. This research team developed an international collaboration that was the first to attempt to move the complete nitrogenase pathway into plant organelles.
The transfer of the pathway had not been previously attempted due to limitations in technology for plant genetic engineering. The DNA encoding the microbial pathway is large (20-40,000 bp) and this is technically challenging to move into a plant (albeit not impossible). The problem is that the genes are under tight regulation in their native host and this does not transfer to plants – so the transfer of the native DNA sequence would not lead to gene expression. Normally, one would replace the microbial regulation with that which would be functional in a plant. However, the model nitrogenase pathway (from Klebsiella oxytoca) requires 16 genes and even slightly misexpression causes a loss in activity. For plant chromosomes, the parts (promoters, etc.) for precision expression control do not exist.
The transfer to a plant organelle would be ideal. Chloroplasts are ancient bacterial systems and they have similar gene regulation. Chloroplasts (at night) have low oxygen tensions, they generate the required energy (ATP) and have appropriate redox capability. Genetic parts that function in bacteria with well-developed tools, such as E. coli, also function in plastid DNA. However, relatively few labs that specialize in engineering these organelles and none of the large agriculture companies are active in this area. The project’s research team at MIT has overcome several critical technical hurdles. The first is that they have reengineered the 16 gene nitrogenase pathway from Klebsiella to systematically remove all of the native regulation and replace it with well-characterized synthetic genetic parts. This modularizes the system such that alternative parts can be rapidly swapped for different target organisms. This pathway is activated by the phage-derived T7 RNA polymerase. They were the first to build a nif pathway using chemical DNA synthesis. They also established the MIT- Broad Foundry and this has become the largest DNA assembly facility in the world. They used this capability to build hundreds of nitrogenase pathways and this resource enabled them to take many “shots on goal.” This project met three goals: 1. Developed genetic parts required for expression in these systems, 2. Designed and built combinatorial nitrogenase pathways for expression in plastids and mitochondria, and 3. Screened for activity. This was the first transfer of nitrogenase activity to a eukaryote.
Outcomes
- Successfully transferred for the first time a functioning nitrogenase pathway into a plant
- Demonstrated nitrogenase activity in plastids and successfully created a synthetic plastid genome using chemical DNA synthesis
- Demonstrated the formation of NifDK tetramers in eukaryotic mitochondria for the first time, signaling that there was active assembly of nitrogenase pathways
- Demonstrated nitrogenase activity in a microbe within the roots of a cereal crop for the first time
Publications
Formation of nitrogenase NifDK tetramers in the mitochondria of Saccharomyces cerevisiae
Stefan Burén, Eric M. Young, Elizabeth A. Sweeny, Gema Lopez-Torrejón, Marcel Veldhuizen, Christopher A. Voigt, and Luis M. Rubio, ACS Synthetic Biology, 2017
News
Additional Details
Impact Areas
- Food
Research Themes
- Sustainability & Adaptation
- Soil Fertility & Crop Productivity
- Transforming Food Systems
Year Funded
- 2015
Grant Type
- Seed Grant
Status
- Completed