Our Research Evaluation of fully synthetic nitrogen fixation pathways, designed for plant mitochondria and plastids
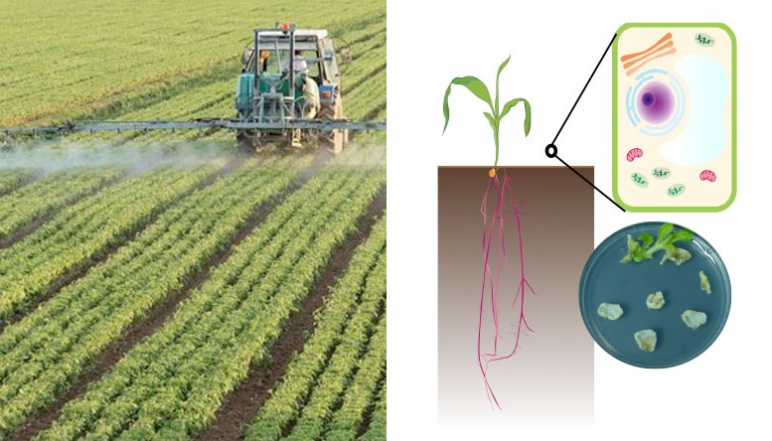
Principal Investigator
Christopher Voigt
- Daniel I.C. Wang Professor of Advanced Biotechnology
- and department head
- Department of Biological Engineering
Christopher Voigt, PhD is the head of the Department of Biological Engineering at MIT. He is an expert in synthetic biology and his lab has applied genetic engineering to problems in agriculture, health, computation, medicine, chemicals/materials, national security, and infrastructure. He holds the Daniel I.C. Wang Professorship and is Co-Director of the Synthetic Biology Center. He received a BSE in chemical engineering from the University of Michigan (1998) and a PhD in biophysics from Caltech (2002). He is a founder of Pivot Bio (microbial agricultural products), Asimov (human cell synthetic biology), and Fieldstone (national security and biotechnology).
Challenge:
How can we enhance the yield of cereal grains without the use of chemical fertilizer?
Research Strategy
- Engineer a method to biologically transfer the nitrogen-fixing capability of legumes to cereal grains via re-programmed plant cell mitochondria
- Initiate an international collaboration of leading scientists (Spain, Germany, and MIT) to speed discovery and development
Project description
Nitrogen, in the form of fertilizer, is required for high agricultural productivity. Primary food crops, like rice, corn or wheat need chemically derived nitrogenous fertilizer. The aim of this team’s first J-WAFS grant was to move the complete microbial nitrogen fixation pathway into chloroplast and mitochondria, since these organelles have similar gene regulation and they make the necessary cofactors. As such, cereals would not require added nitrogen. The transfer of the pathway has not been previously attempted due to limitations in technology for plant genetic engineering. The DNA encoding the microbial pathway is large (20-40,000 bp) and this is technically challenging to move into a plant. Additionally, the genes are under tight regulation in their native host and this does not transfer to plants – so the transfer of the native DNA sequence would not lead to gene expression. Based on J-WAFS funding, the international collaboration with the leaders in plastid engineering (Ralph Bock, Germany) and nitrogenase biochemistry (Luis Rubio, Spain) allowed this team to complete the first plant containing all of the essential nitrogenase genes. An entire nitrogenase cluster was integrated into the plastid genome, which is not functional yet in the plant. For mitochondrial expression, the MIT-Broad Foundary constructed 96 yeast strains containing ~1MB of nitrogenase clusters. From this, they identified a variant that forms complete NifDK tetramers, an essential step of nitrogenase assembly, in the mitochondrion. However, the purified NifDK protein resulted in inactive nitrogenase.
Now that these pipelines for transfer of larger genetic cluster into plant organelles have been established, the renewal of the grant will focus on new designs to obtain nitrogenase activity in these organelles. Three types of new nitrogen fixation clusters are being integrated into plastids: 1. Larger natural clusters with advantageous features (robustness, e- source for nitrogenase, oxygen resistance), 2. Inducible “refactored” cluster with improved stability, and 3. Variants where genetic parts from plastids are substituted into the microbial clusters. Their expression in plants is ensured by ‘omics’ analysis and their functionality is being tested by acetylene reduction assay and nitrogen limited growth experiments. For nitrogenase delivery into yeast mitochondria, several biochemical needs identified from the first library are being incorporated into the next round of constructs to achieve functional nitrogenase assembly in mitochondria. This includes libraries that incorporate additional accessary genes that were omitted from the first library and match the necessary stoichiometry of nitrogenase genes using newly characterized yeast expression cassettes. The research team is biochemically evaluating these libraries for the formation of the active cofactor in the mitochondrion (and intermediates) and activity. These approaches enable the team to take many “shots on goal” in establishing the necessary biochemistry and tuning to optimize the probability of transferring the functional nitrogenase into plants.
Outcomes
- Determined there was a strong correlation between expression of RNA in chloroplast cell-free systems and chloroplast in vivo data in Klebsiella ‘refactored’ v2.1, confirming that the cell-free system can be used to test multi-gene constructs
- Developed chloroplast cell-free system to test and optimize expression of multi-gene constructs before embarking on plasmid transfer, a tedious process
- Detected expression of nif clusters at mRNA level in plastids
- Produced functional NifB, a protein involved in assembling the active-site cofactor for nif gene expression, in aerobically-cultured yeast to avoid issues of toxicity that can arise when E. coli is used
Publications
Formation of nitrogenase NifDK tetramers in the mitochondria of Saccharomyces cerevisiae
Stefan Burén, Eric M. Young, Elizabeth A. Sweeny, Gema Lopez-Torrejón, Marcel Veldhuizen, Christopher A. Voigt, and Luis M. Rubio, ACS Synthetic Biology, 2017
News
Additional Details
Impact Areas
- Food
Research Themes
- Sustainability & Adaptation
- Soil Fertility & Crop Productivity
- Transforming Food Systems
Year Funded
- 2017
Grant Type
- Seed Grant
Status
- Completed